
Composites
Composites are a versatile material that create very strong structures comparable to their metal alloy counterparts,
but at a lighter weight. Composite construction utilizes a weaved cloth made of special fibers that is encased in resin.
Parts can be formed into complex shapes using molds or by covering core materials. In the Homebuilt world
composites are widely used for making anything from small parts to entire airframes. An experimental composite
aircraft usually brings to mind a sleek aircraft capable of high speeds, which is accurate, but composites have many
other applications too.
Composites have been around many years but arrived in the homebuilt world in the 1970’s when Ken Rand and Burt Rutan introduced their respective aircraft designs that utilized composite construction. In the 1980’s these, and other designs became available in kit form to the consumer with pre-molded components. Some of these are still available today on the used market with many new designs added.
Composite materials are generally known as fiber-reinforced plastics which is a plastic resin reinforced with various sheet fibers. The common fibers are fiberglass, Kevlar and carbon fiber. Fibers are soaked and encased in a hardening resin. Multiple layers of fiber sheets can be layered for increased strength. Molds can be used to lay fiber cloth over which is then wet with resin. Vacuum bagging of these molded parts increases the strength and reduces the weight by removing air and excess resin. Foam or wood can be shaped then covered with a composite material to make strong and light structures.
A brief description of the materials follows.
​
Fiberglass
​
Fiberglass is a strong lightweight composite material that is less costly than carbon fiber or Kevlar and is great for molding into complex shapes. Fiberglass is the most well-known of the composites because of its wide use on other products such as boats. Although it is neither as strong nor as stiff when compared to carbon fiber, it is less brittle and its raw materials are much cheaper. Its bulk strength and weight ratios are also better than many metals, and it can more easily be molded into complex shapes than stiffer materials.
E-glass is a standard fiberglass cloth. S-glass is 30% stronger and 15% stiffer than E-glass and retains these properties up to 1500° F. S-glass is also considerably tougher than an equivalent E-glass part.
Carbon fiber
​
As its name implies, carbon fiber fabric is made from carbon fibers. Carbon fiber creates strong, stiff and lightweight parts. Although it is quite costly, a saving of material can offset that to some extent since only one layer of carbon fiber may be required to 3 or 4 of fiberglass.
Carbon fiber comes in several different weaves optimized for different uses. Standard twill weave is a good all-purpose fabric while unidirectional fabric adds strength and stiffness in one direction. Plain weave is good for delicate layups.
Carbon fiber can achieve one of the strongest and most rigid composite structures available. It has significant weight savings when compared to metals and has excellent fatigue resistance.
Kevlar
​
Kevlar has incredible strength, is lightweight, and less dense and far less stiff than carbon fiber and shows no degradation in jet fuel, lubricating oils, water or high humidity.
Kevlar offers a significant weight saving and improved stiffness over fiberglass and also has superior vibration damping. Parts can be close to half the weight of fiberglass and it has a high impact resistance. Kevlar also dampens sound. Cowlings made of Kevlar will be quieter and less sensitive to engine vibrations than its glass or carbon counterparts.
Although all of the resins used with glass fiber are usable with Kevlar, the vinyl ester type system is most compatible. The use of polyesters is not recommended with Kevlar because of poor bonding.
Core Materials
​
Core materials are used to create structures by adhering composite skins over a core material. The most common core materials are wood, aluminum honeycomb, aramid honeycomb, and foam. These can create stronger and stiffer components.
Aircraft grade woods are a readily available core material. Wood can be used to make many components that can be covered with composite materials including wing skins, spars, fuel tanks, and fuselage components.
​
Aluminum honeycomb is a lightweight core material offering excellent strength and good corrosion resistance and low weight.
Aramid Honeycomb is an aerospace-grade aramid fiber honeycomb that exhibits outstanding flammability properties. It is manufactured from Meta-Aramid paper and coated with a heat resistant phenolic resin. Aramid core honeycomb uses include aircraft leading and trailing edges, antennas, fuel tanks and helicopter rotor blades
​
Foam comes in several different types suitable for aircraft components. Foams can be shaped into things like wing leading edges, or sandwiched for fuselage parts. Different types of foam have different properties and applications for aircraft construction.
Polyurethane foam is easily shaped using a large knife then sands well creating your final form. It is excellent for producing fuselage and wing components and other curved parts. It is fuel resistant and can be used for fuel cells and is compatible with most adhesives.
​
Divinycell foam is a closed cell medium to high density foam with high compression strength, durability, and excellent fire resistance. It can be vacuum formed into compound shapes and can be bent and molded with heat. It is compatible with polyester, vinylester, and epoxy resins.
​
Polyether Polyurethane foam (LAST-A-FOAM®) has a fine closed-cell structure and is very versatile for sandwich-core applications. It cuts and shapes easily with common woodworking tools, and bonds to itself and other materials with most epoxy, polyester, or urethane-type adhesives. It is unaffected by water, fuels and most solvents, and paint finishes are easily applied. It is frequently used in regular molds after the gel-coat and first two layers of glass are installed.
​
Large Cell Styrofoam cuts easily using a hot wire to airfoil shapes. Compatible with most adhesives, but should be used only with epoxies, not polyester resins. Will dissolve in fuels and solvents. For structural use in building aircraft such as VariEze, Long-EZ, Cozy, etc.
​
Resins
​
Thermoset resins are used to make most composites. They are converted from a liquid to a solid through a process called polymerization, or cross-linking. When used to produce finished goods, thermosetting resins are “cured” by the use of a catalyst, heat or a combination of the two. Once cured, solid thermoset resins cannot be converted back to their original liquid form. Common thermosets are polyester, vinyl ester, epoxy, and polyurethane. They create an irreversible bond.
Thermoplastic resins, on the other hand, are not cross-linked and, so, can be melted, formed, re-melted and re-formed. Thermoplastic resins are characterized by materials such as ABS, polyethylene, polystyrene, and polycarbonate. These resins are not covered here due to limited use in general Homebuilt construction.
​
The most common thermoset resins are listed below.
​
Epoxy resins have a well-established record for use in a wide range of composite parts and are the resins most often used in Homebuilt aircraft. Epoxies differ from polyesters and vinyl esters in that they harden through a process called crosslinking and form a strong matrix of crosslinked chains. This creates a structural strength within the resin. When combined with the proper reinforcement material, composite structures using epoxies are unmatched in strength and light weight. Like other resins, epoxies are a two-part process consisting of a resin and hardener. Unlike polyesters and vinyl esters, the resin to hardener ratio must be closely followed in epoxies to limit the possibility of undesirable cure characteristics. Adding more hardener will not accelerate the cure time as one may suppose, but may seriously impede the drying and strength of the finished product. Working time with epoxies can be much longer than polyester and vinyl ester since you can use specific hardeners with custom working times with some as short as 4 minutes others over 24 hours. Most epoxy cures at room temperature and once this is complete additional strength can be obtainable by raising the temperature of the epoxy during a "post curing." Usually this involves raising the temperature above 140 degrees Fahrenheit for a period of time. If post curing is not done in the shop, it can still be completed by simply having your airplane on a hot ramp during the summer. Epoxies are used primarily for fabricating high performance composites and have superior mechanical properties, resistance to corrosive liquids and environments, superior electrical properties, good performance at elevated temperatures and good adhesion to a substrate material. Epoxy resins do not have good UV resistance so should be covered with a UV blocking finish if exposed.
​
Unsaturated polyester resins are the most widely used resins in the composites industry and represent approximately 75% of the total resins. They have almost unlimited usefulness in all segments of composite fabrication due to their ease of handling, good balance of mechanical, electrical and chemical properties, and relatively low cost. polyesters adapt well to a range of fabrication processes and are most commonly used in open-molds, spray-ups, compression molding, resin transfer molding and casting. The properties of polyester formulations can be modified to meet specific performance criteria
​
Vinyl ester resins were developed to combine the advantages of epoxy resins but with better handling and faster cure times. Vinyl esters are cured with the conventional organic peroxides used with polyester resins. Vinyl esters offer mechanical toughness and excellent corrosion resistance properties without complex processing, handling or special shop practices. Vinyl ester has good resistance to water and chemically corrosive environments and is good in structural laminates that require a high degree of moisture resistance.
​
Phenolic resin use in composite fabrication has many desirable qualities including high temperature resistance, creep resistance, excellent thermal insulation, sound damping properties and corrosion resistance. However, phenolics polymerize by means of a condensation reaction, which causes the release of water vapor and formaldehyde during cure phase. This can produce voids in the composite part. As a result, phenolic resins mechanical properties are somewhat lower than those of epoxies and most other high-performance resins.
​
​
Vacuum bagging
​
Vacuum bagging, as the name implies, uses a vacuum pump and bagging film to create pressure on the laminate part during the resin cure phase which removes air and excess resin from the part. Pressurizing a composite lamination removes trapped air between layers, it compacts the fiber layers for efficient force transmission among fiber bundles and prevents shifting of fiber orientation during cure. Vacuum bagging most importantly optimizes the fiber-to-resin ratio in the composite part. This maximizes the physical properties of advanced composite parts. The key to obtaining the advantages of vacuum bagging is by maximizing the fiber-to-resin ratio. Fabrics are not particularly strong by themselves and resins are brittle without fabric reinforcement. If there is excess resin in the laminate, the laminate will have more of the properties of resin. If too little resin exists, places where the reinforcement fabric is dry will be weak spots in the part. To optimize the resin content, the entire reinforcement must be saturated with resin with as little excess as possible. Vacuum bagging squeezes out excess resin in order to obtain a maximized fiber-to-resin content. The pros creating refined aerospace composite parts can obtain as little as 40%, but a good target for the rest of us is 60% resin.
​
​

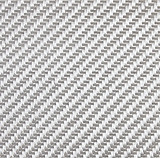

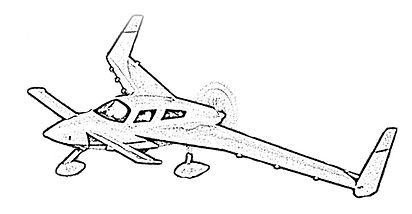